Site Content:
2D Materials and Quantum Devices Group (Stampfer group)
Our research is driven by the vision of “turning quantum properties into functions”. The main systems under investigation are devices based on van der Waals heterostructures formed by graphene and other 2D materials – a unique and still widely unexplored playground for studying quantum phenomena in solid-state systems.
Our research includes the development of advanced process technologies, low-temperature quantum transport and optical spectroscopy techniques. Our activities are shared between the Physics Department in Aachen, and the Research Center Jülich.
We are member of the Aachen Graphene & 2D Materials Center and of the Jülich-Aachen Research Alliance for Future Information Technologies (JARA-FIT), and we host the Junior Principal Investigator Group of Dr. Annika Kurzmann.
Current research activities:
Advanced stacking technologies
In recent years it was shown that the full technological potential of 2D materials is only achieved by stacking them into so-called heterostructures. The interaction between different 2D materials not only can significantly increase the charge and spin transport properties of individual 2D materials, but can lead to completely novel physical phenomena.
The vision is to use such heterostructures to realize e.g. spin- or valley-based electronics or highly sensitive sensors based on these novel effects. However, current fabrication technologies for such heterostructures lack two aspects of great technological importance: reproducibility and scalability. We therefore investigate advanced stacking technologies to address these problems. One issue is e.g. the mere exposure of 2D materials to air, as this leads to the deposition of airborne hydrocarbons onto their surfaces, which diminishes the quality of heterostructures in an uncontrolled manner. We are therefore building a transfer system inside an ultrahigh-vacuum chamber, which should allow us to achieve atomically clean interfaces between different 2D materials over large areas. Furthermore, we are continuously optimizing traditional stacking techniques under both ambient conditions and under the inert gas atmosphere of a glovebox system, to improve e.g. our control of the alignment between different 2D materials.
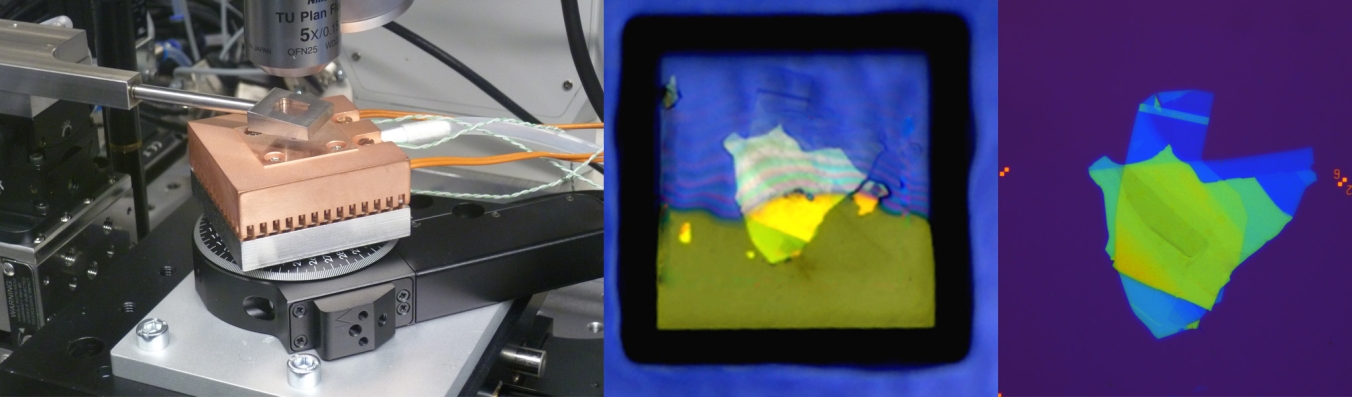
•Advanced stacking technologies
Boron nitride benchmarking
The pathway to both scalable growth and transfer methods for 2D materials is one of the key challenges to make the leap from laboratory to industry. Of particular importance is the material (hexagonal) boron nitride, which has proven to be an excellent insulating substrate and protective layer for graphene, 2D semiconductors, metals, magnets and superconductors. To date, graphene/boron nitride structures allow for record charge carrier mobilities at room temperature but are limited to individual devices.
Within the graphene flagship consortium of the EU, we characterize boron nitride crystals and large scale thin films transferred by different methods onto the target substrate. The quality of these materials are investigated using atomic force microscopy and Raman spectroscopy. To further test the structural and electronic quality of the different materials, we use graphene as a sensitive sensor. For this purpose, graphene gets fully encapsulated into boron nitride using dry transfer methods. Hall-bar structures are then processed, and the electron transport properties are measured both at cryogenic temperatures and at room temperature. Based on these measurements, we aim to better understand the influence of different growth parameters on the quality and the device-to-device reproducibility we are ultimately interested in.
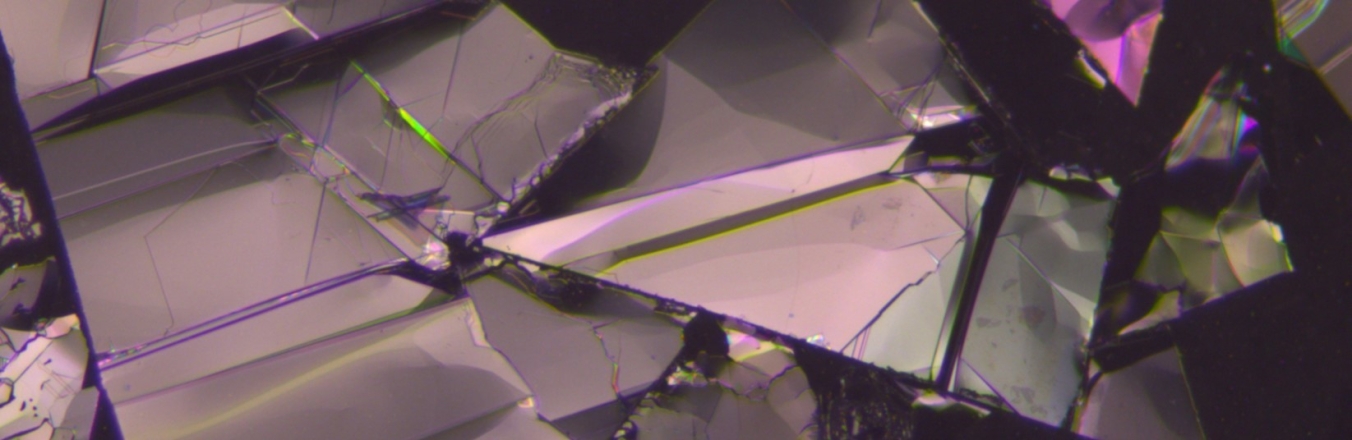
•Boron nitride benchmarking
High quality CVD Graphene
Graphene has numerous properties such as its optical transparency, excellent electrical conductivity and remarkable mechanical properties that make it well suited for a large variety of potential applications. Amongst the most promising applications are high-frequency electronics, transparent electrodes and ultra-precise sensors for magnetic fields. For any of these applications to be realized, it is crucial to high quality graphene for a reasonable price. We work on large area graphene growth using chemical vapor deposition (CVD) on copper and on a dry and contamination-free process that allows to transfer the graphene on any substrate, while preserving its very high electronic quality. In order to reduce the cost of production for graphene, the copper substrate can be reused for multiple growth cycles.
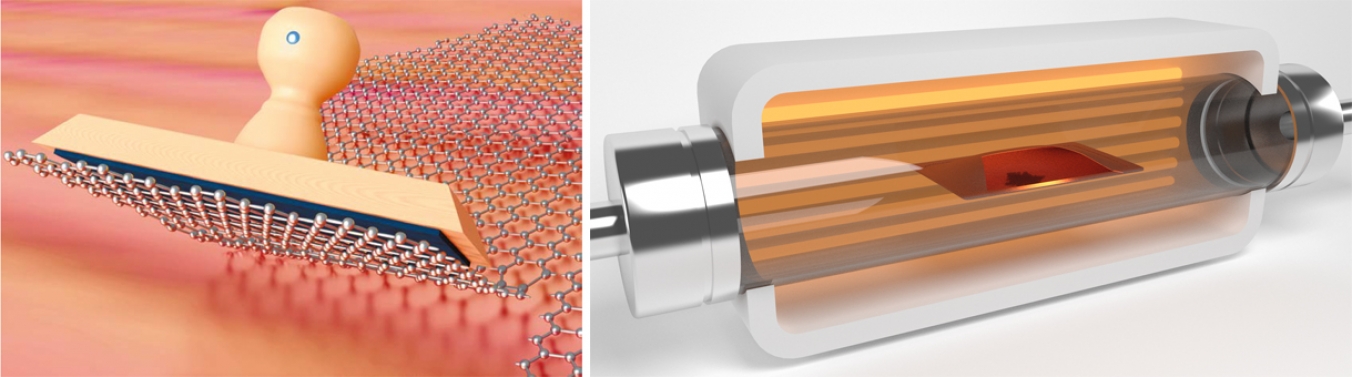
•High quality CVD Graphene
Electrical spin transport in graphene-based heterostructures
One of the long-term objectives of our research is to incorporate the spin degree of freedom of charge carriers into electronic devices. This enables a completely new class of electronics, so-called spintronics. In recent years, two-dimensional materials like graphene or monolayers of transition metal dichalcogenides (TMDs) have shown to have the potential to outperform conventional spintronic materials such as silicon and gallium arsenide, especially in the technological relevant temperature range near room temperature. Here, graphene is an ideal material for the transport of spin information as it has low spin-orbit coupling, weak hyperfine interaction, and high charge carrier mobilities. This keeps electron spins coherent across micrometer long length scales even at room temperature. However, all the properties of graphene that protect the spins are a double-edged sword, as the manipulation of spins is being limited at the same time. Therefore, our research effort lies in a modification of graphene’s spin transport properties by e.g. proximity-induced spin orbit coupling introduced by stacking graphene and transition metal dichalcogenides into heterostructures. Moreover, our research activities also focus on the understanding of various mechanisms behind spin dephasing and spin relaxation and the investigation of (quasi-)ballistic spin transport.
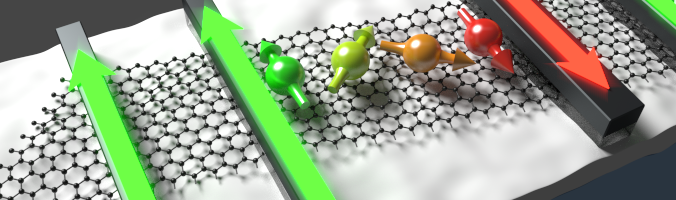
•Electrical spin transport in graphene-based heterostructures
Electrostatically-defined bilayer graphene qantum dots
Graphene is considered a promising material for quantum information applications, since its low spin-orbit interaction and low hyperfine coupling suggests long spin and valley coherence times. We aim to build a qubit using the spin- or valley degree of freedom of a single charge carrier confined in a quantum dot in bilayer graphene.
Bilayer graphene has the unique property that a perpendicular electric field opens up a band gap. Fabricating devices with elaborate gate structures as shown below, we are able to confine single charge carriers in quantum dots by locally applying a perpendicular electric field. Our samples are highly tunable due to different layers of metallic gates, allowing to create quantum dots of opposite polarity, chosen occupation number and desired tunnel coupling in close proximity [1,2,3].
We then perform transport measurements to evaluate the single- and two-particle spectrum [4,5] and RF-pulse-gate measurements to investigate the dynamics of these states. Further measurements to manipulate selected quantum dot states are planned.
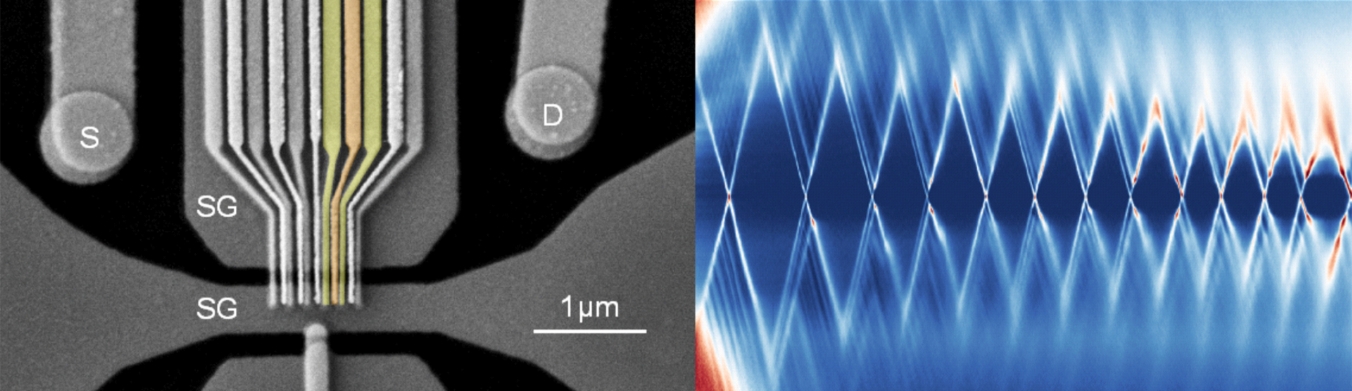
•Electrostatically-defined bilayer graphene qantum dots
Quantum point contacts in bilayer graphene
Bernal stacked bilayer graphene (BLG) offers the unique possibility to open and tune a band gap by applying a perpendicular electric field. Experimentally, an electric field can be applied by placing the BLG between a top and bottom gate, allowing the Fermi level within the BLG to be changed independently of the band gap. By combining the high control capabilities of dual gates with multiple sets of gates, BLG can be electrostatically divided into insulating and conducting parts. Thus, it is possible to implement a soft-confined one-dimensional channel and create BLG quantum point contacts (QPC).
In BLG QPCs, we can control the Fermi-Level, the confining potential, and the polarity of the charge carriers, which makes them an ideal platform for studying the spin- and valley-dependent properties in BLG. We are able to measure the very small spin-orbit gap in BLG (around 40 microelectronvolt) and its dependence on the applied displacement field. Moreover it is possible to measure valley polarized transport or a tunable valley splitting. Currently, we are working on utilizing BLG QPCs as spin or valley filter by combining QPCs of different channel width.
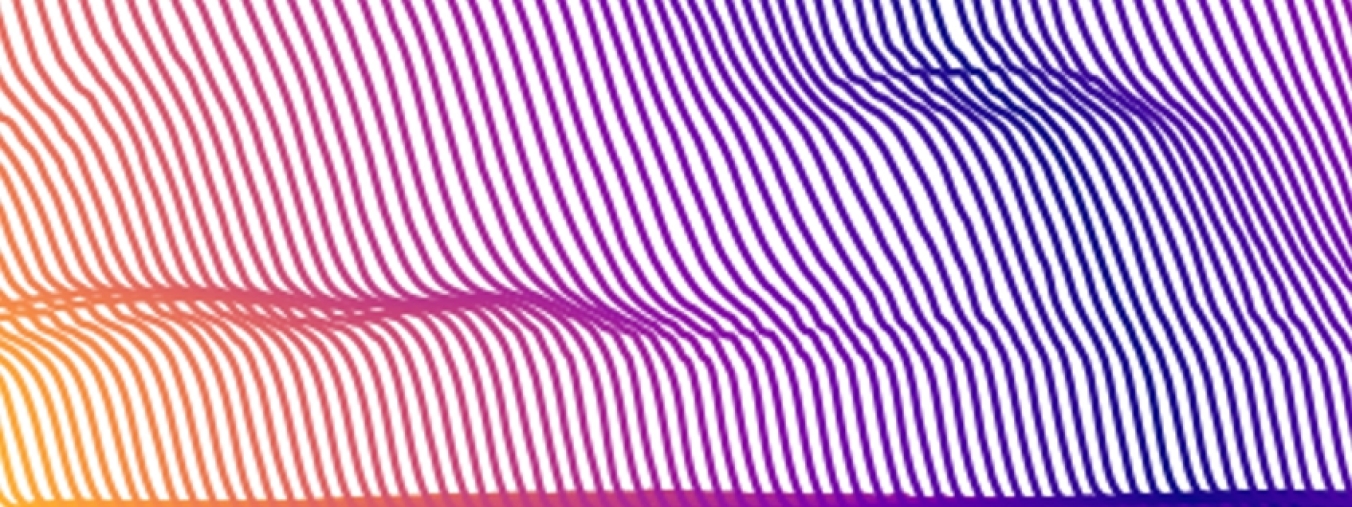
•Quantum point contacts in bilayer graphene
Raman spectroscopy and transport on twisted bilayer graphene
When two layers of graphene are stacked with a twist angle, a moiré pattern will form between the two layers. The electronic properties of this material are highly sensitive to the twist angle, allowing one to tune how fast electrons can travel in this material. Near the so-called “magic angle” of 1.1˚, the electrons slow so much that unusual electronic phenomena arise, such as superconductivity and correlated insulating states. The exact nature of these states is still a debated subject, but understanding them may bring us closer to understanding exotic phenomena such as high-temperature superconductivity.
One problem with twisted bilayer graphene is that the twist angle may vary over the sample area, which affects the electronic transport properties. Our group focuses on Raman spectroscopy as a means to image the twist angle variations over a sample. By using a laser focused on the sample, we can detect specific vibrations in the atomic lattice that only occur in the twisted material. By measuring the frequencies of these vibration, we can map out tiny variations in the twist angle of our samples.
Furthermore, we are interested in understanding the insulating states that emerge in this material, and how we can build devices that have the largest possible electrical resistance. This will allow us to build higher quality devices with new functionality.
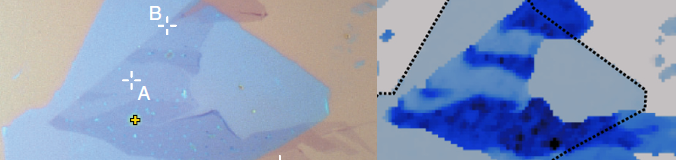
•Raman spectroscopy and transport on twisted bilayer graphene
Opto-electronic properties of 2D semiconductors and their heterostructures
Monolayers of transition metal dichalcogenides have fascinating properties: they are mechanically flexible, air-stable semiconductors which exhibit particularly strong light-matter interactions and have potential applications from miniaturized LEDs or solar panels to novel concepts of information processing.
Their two-dimensional (2D) structure has many interesting effects compared to classical (3D) semiconductors. For example, the screening of Coulomb-interactions is geometrically reduced as field-lines extend outside the material, leading to the appearance of strongly bound excitonic and many-body states and even excitonic Bose-Einstein condensates. Moreover, the strength of the Coulomb-interactions within the 2D semiconductors is influenced by their environment (which adds to the screening), allowing for the manipulation of their opto-electronic properties in a completely novel way.
We use and develop optical spectroscopy methods to study two-dimensional semiconductors and employ a wide range of techniques to manipulate their electronic structure. This includes electrostatic fields for charge doping, dielectric screening by the environment, magnetic proximity-effects and pulsed optical excitations, which allow studying the temporal dynamics of carriers and spins.
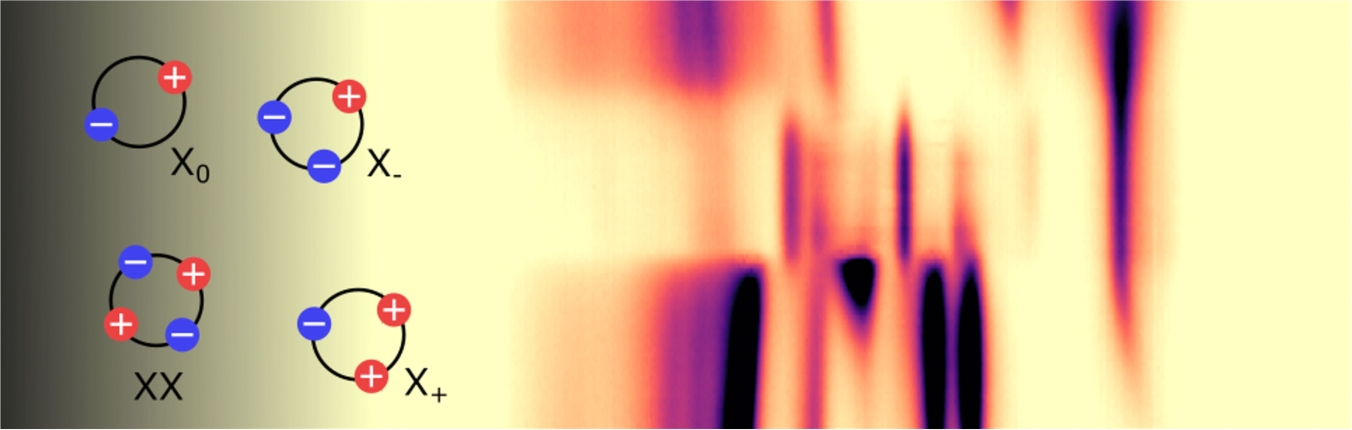
•Opto-electronic properties of 2D semiconductors and their heterostructures
Spin and valley dynamics in heterostructures of TMDs
Monolayers of transition metal dichalcogenides (TMDs) are direct band gap semiconductors with band gap energies that lie either in the optical range of the electromagnetic spectrum or the near-infrared. Furthermore, TMDs have the unique feature of a large valley-dependent spin-orbit splitting. These two features enable the valley-selective optical excitation of spins in these materials. Therefore, the combination of TMDs with graphene and hexagonal boron nitride enables us to fabricate advanced van-der-Waals heterostructures for devices that couple optics with spin-sensitive electronics (so-called spintronics).
We are especially interested in investigating the valley- and spin-dynamics in such heterostructures in dependence on parameters like gate-induced charge carrier densities or proximity-induced effects to other 2d materials like ones that exhibit ferromagnetism. For the experiments we employ a wide variety of optical and electrical measurements techniques, e.g. time resolved Kerr rotation, photoluminescence, reflection and absorption spectroscopy, or photo-current measurements.
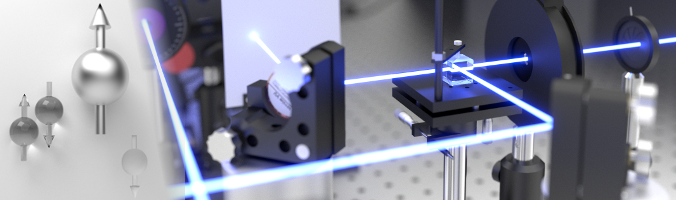
•Spin and valley dynamics in heterostructures of TMDs
Graphene Quantum Electromechanical Systems
The Extraordinary mechanical and electromechanichal properties of graphene – truly 2d material – make it one of the best candidate for future ultra-high frequency resonators, nano sensors or radio transducers. A detailed understanding of the interplay between mechanical and electronic properties of graphene is of high importance. For this reason a complete separation of mechanical degrees of freedom from all other adjustable parameters - i.e. temperature, charge carrier density, external pressure etc. - is considered as an important step towards exploring the fundamental properties of graphene nano-electromechanical systems. We are using an integrated system, where a graphene flake is transferred over a suspended movable silicon-based comb-drive actuator. The comb-drives are designed such that they can induce significant mechanical forces to strain graphene and the amount of strain is controlled independently. By using highly doped silicon the integrated comb-drive devices can be actuated at cryogenic temperatures, where a higher energy resolution will provide potentially more insights into the fundamental electromechanical properties of graphene. Further device functionality is introduced by a local bottom gate that enables to control the carrier concentration in graphene by tuning the Fermi level.
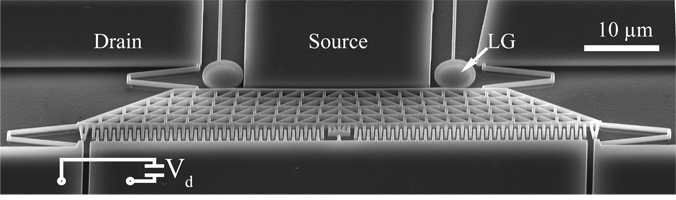